2016 Acs Quantitative Analysis Exam Study Guide
Amendments were made to this protocol on February 15, 2017. To view the amendments, go to. Background and Objectives for the Systematic Review Heart disease is the leading cause of death worldwide. 1 In the United States, where it is the leading cause of death for both men and women, heart disease accounts for more than 600,000 deaths annually, or 23.5% of deaths from all causes.
2 Over 25 million adults in the United States are currently estimated to be living with a diagnosis of heart disease, 3 and over 1 million people in the United States are estimated to be hospitalized for an acute coronary syndrome i.e. Unstable angina pectoris or myocardial infarction (MI) each year.
4 Patients who are diagnosed with acute coronary syndrome (ACS) are at risk for a range of negative health outcomes. For the purpose of this review, ACS refers to clinical symptoms compatible with acute myocardial ischemia and includes unstable angina (UA), non-ST-segment elevation myocardial infarction (NSTEMI), and ST-segment elevation myocardial infarction (STEMI).
Post-ACS patients may be at greater risk for mental health problems—in particular, depression. 5 In the general population, lifetime prevalence of depression symptoms is approximately 17%, 6 studies however have found that as many as 65% of post-MI patients experience symptoms of depression. 7,8 Depressive disorders are characterized by persistent depressed mood or anhedonia, other associated symptoms such as sleep disturbance or decreased energy, and functional impairment for at least 2 weeks. Major depressive disorder (MDD), persistent depressive disorder (DSM-IV dysthymia), and subsyndromal depression are highly prevalent in general medical populations (2-16% within the US) 9-13 and are estimated as the second largest cause of loss in disability-adjusted life years. 14 Depressive disorders are associated with chronic medical illness, including cardiovascular disease, and worse general medical outcomes.

Patients with depression post-MI have significantly increased risk of death. 15 Despite the high prevalence of depression, the association with cardiovascular disease, and the profound impact on quality of life (QOL), there is considerable uncertainty about whether and how to screen patients for depression post-ACS.
Guidelines for screening for depression in primary care settings vary. The 2016 guidelines from the U.S. Preventive Services Task Force (USPSTF) recommend that depression screening be 'implemented with adequate systems in place to ensure accurate diagnosis, effective treatment, and appropriate follow up.' 16 However, other guidelines recommend targeted screening for patients at increased risk of depression 17 or against routine screening. 18 Individuals post-ACS are at higher risk for depression and some professional societies recommend routine screening during and after the post-MI hospitalization, but these guidelines are controversial. 19,20 It is unclear how well standard instruments for detecting depression perform in this medically ill group and whether this group would benefit from targeted screening. It is also unclear whether post-ACS patients with depression respond any differently than people in the general population with depression to commonly used, efficacious treatments for depression.
Such treatments include pharmacotherapy and psychotherapy, with second generation antidepressants and cognitive behavioral therapy (CBT) being among the most widely supported, evidence-based, depression treatment approaches. Both pharmacotherapy and psychotherapy have been shown to be effective, 15 although it is unclear whether combination therapy is superior to pharmaco- or psychotherapy alone.
It is possible, though not clearly established, that some of these treatments for depression may function differently in post-MI patients. 21 For instance, behavioral activation, a core component of many CBT-based approaches, might encourage the adoption of new behavioral repertoires that not only improve mood but also medical outcomes. 22 The same could be hypothesized about exercise, which has been demonstrated to have beneficial effects for emotional health 23 and cardiovascular health. 24 Alternatively, it may be that certain depression treatments that are usually effective in the general population are less so among post-ACS patients, or carry certain risks that might be of particular concern in this population. 21 Depression treatments include antidepressant medications, adjunctive medications (e.g., atypical antipsychotics), psychotherapies, light therapy, supportive strategies (e.g., exercise, guided self-help), and combinations of these approaches. In addition, collaborative care, a method to improve care delivery, is a potential intervention of interest.
For this review, we have expanded the scope to consider all patients with ACS (unstable angina and MI), criterion-based (DSM-V or equivalent) depressive disorders, and treatment approaches prioritized by the stakeholders and Technical Expert Panel. Given available resources and discussions with both the nominator and additional stakeholders, we will not include patients who are post-PCI or post-CABG. Discussion of the impact of this decision will be included in the report. Information on the FDA status and warnings for use the medications considered in this review are provided in Key Questions The draft key questions (KQs) developed during Topic Refinement were available for public comment from May 26, 2016 to June 15, 2016. Overall, the comments affirmed our planned approach. Specific suggestions included: (a) clarifying the screening tools to be considered and how International Classification of Diseases (ICD) diagnoses would be used in the context of a validated criterion standard, (b) adding transcranial magnetic stimulation, (c) clarifying aspects of the adverse effects to be considered, such as distinguishing between treatment discontinuation due to adverse effects vs efficacy and further distinguishing among suicide-related behaviors, and (d) clarifying other specific outcome elements. Revisions made in response include: clarifying the screening tools and adding the Quick Inventory of Depressive Symptomatology to the interventions of interest in KQ 1, clarifying handling of ICD diagnoses, adding transcranial magnetic stimulation as an intervention of interest for KQ 2, and clarifying adverse effects outcomes.
Additional modifications to outcomes included explicitly adding remission within the category of depression-related outcomes and specifying the types of emergency room visits of interest. There were no other significant changes to the KQs or proposed methods. Note that the scope of the review does not explicitly address the linkage between the use of screening tools in KQ1 and downstream clinical outcomes.
This limitation in scope will be addressed in the discussion of the findings and highlighted as an area for potential future research. Also note that although ease of use and user burden are not listed within KQ1 as specific outcomes of interest, the systematic review will include a summary table of the screening tool characteristics (e.g., number of items, availability/fees) to aid in the comparison and intepretation of our findings.
KQ 1 What is the accuracy of depression screening instruments or screening strategies compared to a validated criterion standard for post-acute coronary syndrome (ACS). patients?
Filter aided sample preparation (FASP) and related methods gain increasing popularity for proteomic sample preparation. Nevertheless, the originally published FASP method has been criticized by several authors, who reported low digestion performance. In this work, we re-evaluate FASP and the related multienzyme digestion (MED) FASP method. We use different types of animal tissues and cultured cells and test the performance of the method under various conditions. We analyze the protein to peptide conversion by assessing the yield of peptides, frequency of peptides with missed cleavage sites, and the reproducibility of FASP. We identify conditions allowing efficient protein processing with high peptide yields and demonstrate advantages of the two step digestion strategy over single step digestion with trypsin.
In addition, we show that FASP outperforms in-solution cleavage strategies. Our results clearly demonstrate that the performance of digestion varies between different types of samples. We show that MED FASP in combination with the total protein approach provides highly reproducible protein abundance values. The presented data can be used as a guide for optimization of sample processing. Sample preparation is the most critical step in the proteomic workflow.
In shotgun bottom-up proteomics, which is the most popular way to analyze proteomes, a large number of different methods for protein extraction and digestion coexists. Besides the original “gel-” and “in-solution” digestion protocols, approaches using solid phase supports or reaction vessels, considered as proteomic reactors, gain increasing popularity. The latter class also includes ultrafiltration based methods that employ centrifugal protein concentrators. These “reactors” allow sample purification, chemical derivatization, and enzymatic digestion.
They enable more flexibility in sample processing, such as successive cleavage, and sample fractionation, which increases depth of proteomics analysis and facilitates identification of low abundant proteins. An additional advantage of the ultrafiltration approaches is the purity of the digests, which is a prerequisite for effective peptide fractionation and mass spectrometry using MuDPIT or isoelectric focusing. Filter aided sample preparation (FASP) and the multiple enzyme digestion FASP (MED FASP) method were developed and successfully applied to the analysis of cultured cells as well as tissue lysates from fresh, frozen, and formalin fixed, paraffin embedded samples. Across different studies using various sample type and sample size, we have reported 50–70% yields of the protein to peptide conversion and low content of peptides with missing cleavages. However, performance of FASP was not tested systematically. In this report, we evaluate the performance of FASP and MED FASP applied to processing of whole lysates samples from mouse organs and human cells. We test the digestion efficiency in terms of quality and yields of peptides using different conditions and sample amounts.
We show also the advantages of the ultrafiltration based digestion protocol compared to in-solution digestion methods. Protein lysates were processed by the FASP and MED FASP protocol using Microcon 30k centrifugal ultrafiltration units (Merck, Darmstadt) operated at 10 000 g. We reduced the centrifugation force below the maximum recommended by manufacturer (14 000 g). This was necessary to circumvent an often observed damage of the ultrafiltration membrane during centrifugation. Aliquots containing 25–400 μg of total protein were mixed with 200 μL of 8 M urea in 0.1 M Tris/HCl, pH 8.5 (UA), in the ultrafiltration unit and then centrifuged at 20 °C, for 15 min.
The eluates were discarded; 100 μL of UA was pipetted into the filtration unit, and the units were centrifuged again. Then, 50 μL of 0.05 M iodoacetamide in UA was added to the filters, and samples were incubated in darkness for 20 min. Filters were washed twice with 100 μL of UA followed by two washes with 100 μL of 0.05 M Tris/HCl, pH 8.5 (digestion buffer DB), containing 0, 1, 2, 3, 4, 6, or 8 M urea and were digested in 40 μL of the buffer used for washing at 37 °C for 18 h, using endoproteinase LysC (MED FASP) or trypsin (FASP), at an enzyme to protein ratio of 1:100. The released peptides were collected by centrifugation at 10 000 g for 10 min followed by two washes with 100 μL of DB. In the MED FASP protocol, the material remaining on the filter was digested with trypsin using the above conditions, except that the cleavage reaction was performed only for 2 h.
The eluates containing 10 μg of total peptide were desalted on C 18-StageTips and concentrated to a volume of 4–5 μL and were stored frozen at −20 °C until mass spectrometric analysis. Twenty μL aliquots of SDS lysates containing 100 μg of total protein were mixed with 80 μL of absolute ethanol and incubated at −80 °C for 3 h.
The precipitate was collected by centrifugation at 10 000 g for 10 min. The resulting pellet was washed with 80% ethanol, vacuum-dried, and dissolved in 25 μL of UA.
Then, 1 μg of LysC was added, and the mixture was incubated at 37 °C overnight. After dilution with 75 μL of DB, the samples were digested with 1 μg of trypsin at 37 °C for 3 h. For determination of the digestion efficiency, the digest was passed through the Microcon 30k to isolate peptides. Analysis of the peptide mixtures was performed with an LTQ Orbitrap instrument (Thermo-Fisher Scientific) as described previously. Briefly, aliquots containing ∼5 μg of peptides were injected and separated on a reverse phase column (20 cm × 75 μm inner diameter) packed with 1.8 μm C18 particles (Dr.
Maisch GmbH, Ammerbuch-Entringen, DE) using a 4 h acetonitrile gradient in 0.1% formic acid at a flow rate of 250 nL/min. The LC was coupled to the mass spectrometer via a nanoelectrospray source (Proxeon Biosystems, now Thermo Fisher Scientific). The LTQ Orbitrap was operated in data dependent mode with survey scans acquired at a resolution of 60 000 at m/ z 400. For CID fragmentation, up to the 10 most abundant precursor ions from the survey scan with charge ≥+2 within the 300–1700 m/ z range were selected. The normalized collision energy was 35.
The dynamic exclusion parameters were 90 s and 5 ppm. The MS 2 spectra were acquired in the ion-trap. The MS data from mouse and human material were analyzed in MaxQuant software. Proteins were identified by searching MS and MS/MS data of peptides against a decoy version of the UniProtKB (May 2013).
Carbamidomethylation of cysteines was set as a fixed modification. N-terminal acetylation and oxidation of methionine were set as variable modifications. Up to two missed cleavages were allowed.
The initial allowed mass deviation of the precursor ion was up to 6 ppm, and for the fragment masses, it was 0.5 Da. The “match between runs” option was enabled to match identifications across samples within a time window of 2 min of the aligned retention times.
The maximum false peptide discovery rate was specified as 0.01. Protein concentrations were calculated by the TPA method. The statistical analysis of the data sets was performed in SigmaPlot12 software. The percentage of partially cleaved peptides (MCPs) was normalized by their spectral intensities. FASP protocols that allow quantitative depletion of SDS and sample digestion were developed several years ago. Since then, the protocols have been optimized for sample preparation efficiency and workload.
First, substitution of the 10kD cutoff Microcon filtration units with 30 kDa cutoff units reduced the time needed for the multiple centrifugation steps by approximately 3-fold. Second, by decreasing of the centrifugation force from 14 000 g to 10 000 g, we eliminated losses of sample due to frequently observed breaking of the filtration membrane (unpublished). Since the frequency of filter breaking has been varying between batches of filters, we attribute the reduction of the ultrafiltration membrane robustness variation in the manufacturing process rather than an effect of a prolonged storage. Third, we reduced the number of solutions used in the procedure to only two: a “cleaning solution” (UA) and a “digestion buffer” (DB). Finally, we found that there are limits in the sample amounts that can be processed at peptide yield and that addition of urea to the digestion mixture has only negligible influence on the FASP efficiency. Moreover, we found that overall performance of the methods depends on the sample type. These observations were derived from several unrelated studies, rendering an overall assessment difficult.
Here, we have benchmarked FASP performance systematically. To test the capacity of the filtration device, we processed different amounts of sample using LysC and trypsin in either FASP or MED FASP formats. Whole tissue lysates of mouse liver and brain were used. The highest yields of the protein to peptide conversion were observed for samples containing up to 100 μg of total protein.
For higher loads, a continuous decrease of yields was observed. For loads of 400 μg of total protein, the peptide yield was halved compared to the 100 μg loads. Similar results were obtained using filtration units with vertically oriented membranes (A).
However, for sample amounts of 100 μg of total protein or less, the peptide yields were considerably reduced compared to the flat bottomed units. Efficiency of protein to peptide conversion at varying amounts of processed total protein. Whole tissue lysates of mouse brain (A) and liver (B) in 2% SDS were processed by MED FASP. Digestions with LysC and trypsin were performed in the absence of urea. The yields were expressed as a ratio of the amount of eluted peptides to total protein amount processed. Black and red dots refer to the first and the second digestion in MED FASP, respectively. The orange dots (MED FASP) are the sum of the yields from both digestions.

The ratio of LysC and trypsin to total protein was 1:100. The experiments were performed in triplicates. Bars indicate standard deviation. Urea is a commonly used reagent in various proteomic sample preparation protocols. In the FASP methods, concentrated urea is used for depletion of SDS, and in the original protocol sample, digestion was carried out in 8 M urea solution. In this study, to test the effect of urea on the digestion efficiency, we processed whole lysates from mouse brain, mouse muscle, and human CaCo-2 cells using buffers containing 0 to 8 M off the denaturant. In the FASP procure, using single step digestion with trypsin, in the presence of 1 or 3 M urea, had only minimal effect on the peptide yield.
At urea concentrations exceeding 4 M, the peptide yields were strongly reduced. A similar relation of urea concentration and digestion yields was observed in both mouse tissues and CaCo-2 lysates. In the MED FASP format, the increasing concentrations of urea were accompanied by a maximal 20% increase of peptide yields during the LysC cleavage. In parallel, the yields of trypsin digestion were decreasing with an increase of urea concentration.
Overall, the sum of the peptides released by LysC and trypsin was changing only a little between 0 and 6 M urea. Efficiency of protein to peptide conversion in the absence or presence of urea. 100 μg aliquots of whole lysates of mouse brain (A) and muscle (B) and human CaCo-2 cells in 2% SDS were processed by FASP ( blue dots) and MED FASP ( orange dots).
The yields were expressed as a ratio of the amount of eluted peptides to total protein amount processed. Black and red dots refer to the first and the second digestion in MED FASP, respectively. The orange dots are the sum of the yields from both digestions.
The peptide mixtures obtained by FASP and MED FASP from brain, muscle, and CaCo-2 cells were analyzed by LC-MS/MS using 4 h gradient chromatography and an “Orbitrap” mass spectrometer. This analysis revealed that the presence of urea in concentrations of 4 or 6 M used during digestion has little effect on the sequencing efficiency of peptides. As a consequence, the number of identified peptides varied only little. However, we observed significant differences between different types of samples. The peptide mixtures obtained from the cultured cells and brain were more frequently sequenced than the peptides from muscle, and this was reflected in the numbers of identified peptides. The LysC and tryptic fractions from MED FASP analyzed separately resulted in fewer peptide identifications than the tryptic peptide fraction generated by FASP. However, when the numbers of unique peptides were summed, their total number increased up to 90% compared to the single tryptic digests.
Acs Quantitative Analysis Exam Review
The larger number of unique peptides results in identification of an additional 30–40% proteins (C,F,I). Identification of unique peptides and proteins by LC-MS/MS using different digestion conditions.
Peptides generated by FASP and MED FASP from whole tissue (A–F) and cell (G–I) lysates were separated by 4 h LC and analyzed with an Orbitrap instrument. “MS2 efficiency” indicates the portion of MS1 spectra resulting in MS2 spectra leading to peptide identification. The “LysC” ( black) and “Trypsin after LysC” ( red) symbols refer to MED FASP digestion steps.
Bars in C, F, and I show the number of identified proteins per two LC-MS/MS, of either tryptic peptide fractions or single MED FASP composed of a LysC and tryptic digests. The bars are averages of data resulting from the analysis of samples digested in 0, 1, and 2 M urea. Shows rates of MCPs found in the analysis of the tissue and cell samples. Across all samples and conditions used, digestion with LysC resulted in the lowest frequency of MCPs ranging from 2% to 4%, in the absence or the presence of urea up to 6 M. At 8 M urea, the rate of missed cleavages increased to 10% in the muscle sample (C). The second step in the MED FASP procedure, the digestion with trypsin, resulted in 5–10% of MCPs in fractions produced in the absence of urea. A continuous increase of the amount of MCPs was observed with increasing concentrations of the denaturant.
The extent of missed cleavages was lower in the CaCo-2 and muscle than in the brain samples. In the past, several reports questioned the effectiveness of FASP and suggested other digestion strategies as better solutions for sample preparation. We compared FASP and MED FASP with an “in-solution” digestion method.
In this comparison, we used either whole brain lysates or microsomal fractions from mouse liver. We found that FASP resulted in 1.5–2 times more peptides than the “in-solution” protocol (A,B). As a consequence of weaker cleavage efficiency, the “in-solution” digests contained about 2-fold more MCPs (C). This comparison shows that the FASP methods outperform the digestion “in-solution”. Notably, a high content of MCPs in digests prepared by “in-solution” methods was also reported previously. Reproducibility of the sample preparation method is a prerequisite for relative and absolute protein quantification.
To evaluate reproducibility of the MED FASP and FASP methods, we compared the concentrations of proteins calculated by TPA between single technical repeats using the Pearson Correlation. We compared concentrations of proteins quantified in all samples, which were identified with at least 3 peptides.
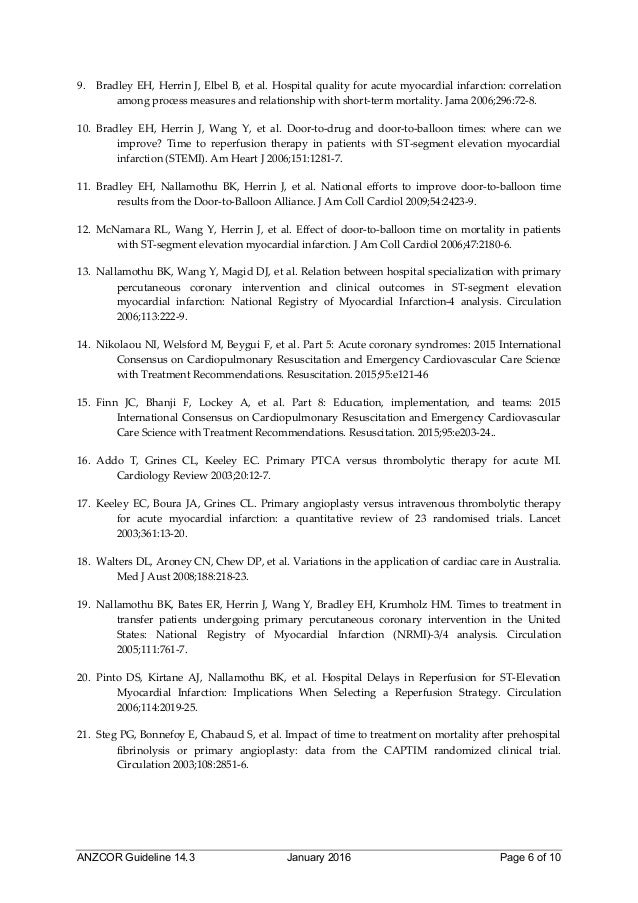
The set of brain lysate analyses comprised 2957 and 1264 proteins for the MED FASP and FASP experiments, respectively (A,B). For all technical repeats, we found an excellent correlation of r ≥ 0.99 between MED FASP runs and ≥0.98 between FASP single measurements (A,B).
Comparison of the data across samples processed at various urea concentrations also showed negligible variation ( r ≥ 0.97) indicating that moderate changes in the denaturant concentrations have practically no effect on the final results. We also analyzed our data using two other label free quantitation (LFQ) approaches, which are used only for relative protein quantitation, the intensity based MaxQuant algorithm, and spectral counting. Whereas the MQ-LFQ data showed similar correlations compared to TPA, the correlations between the data obtained by a spectral counting approach were more variable (C,D). Similarly, a high correlation between single analyses of MED FASP was observed for muscle and CaCo-2 cells (E,F). These results clearly demonstrate the robustness of FASP methods for sample processing. Reproducibility of the MED FASP (A and C–F) and FASP (B) methods. The figure shows the Person Correlation coefficients between the values obtained from the MaxQuant “raw intensity” output processed with the Total Protein Approach (TPA; panels A, B, E, and F), the MaxQuant-“LFQ algorithm” (LFQ; panel C) data, and MaxQuant-“spectral counts” (SPC; panel D) for brain (A–D), muscle (E), and CaCo-2 lysates (F).
Only proteins identified in all single analyses with at least 3 peptides were selected. The data cover duplicate analyses of samples digested in the presence of 0, 1, 2, or 3 M urea. The technical duplicates are boxed. In this study, we re-evaluated the FASP and MED FASP procedures using “Microcon” ultrafiltration units.
Our results reveal the performance of the methods for the analysis of tissue and cultured cells. We determined the limits in protein amount that can be processed and found that digestion in the absence of urea results in peptide mixtures with the lowest rate of MCPs. The presented results indicate that the sample preparation outcome varies between different sample types. In terms of protein to peptide conversion and missed cleavage rates, FASP outperforms the “in-solution” digestion procedure. Our results also emphasize advantages of MED FASP over the single enzyme digestion in terms of quality and depth of proteomic analysis.
It has been well documented that elution of peptides after the first digestion changes the cleavage reaction equilibrium, which in-turn facilitates digestion of less abundant proteins as well as digestion of sites with lower affinity to enzyme. Moreover, reduction of the peptide concentrations by stepwise elution and digestion may reduce an often unnoticed process of transpeptidation, in which already released peptides and amino acids are transferred to another amino compound. Obviously, transpeptidation products escape detection during common data searches.
In this study, we focus on FASP methods applied for total protein amounts larger than 25 μg. We expect that our conclusions are also valid for preparations using smaller sample amounts. For example, in a recently reported application of FASP to low protein level samples, as the secretome from murine Langerhans islets, digestion was conducted in the absence of any denaturant. This is in line with the findings of this work showing that urea worsens rather than improves the performance of protein digestion. The Supporting Information is available free of charge on the at DOI:. Supplementary Table 1, peptides identified by the analysis of mouse brain lysates.
Supplementary Table 2, peptides identified by the analysis of mouse muscle lysates. Supplementary Table 3, peptides identified by the analysis of human CaCo-2 cells. Supplementary Table 4, proteins identified by the analysis of mouse tissue and CaCo-2 cell lysates. Supplementary Table 5, peptides identified by the analysis of mouse brain lysates using an “in-solution” digestion method.
Acknowledgment We thank Prof. Matthias Mann for continuous support, Dr. Georg Borner for critical reading of the manuscript, Katharina Zettl for technical assistance, and Igor Paron and Korbinian Mayr for support in mass spectrometric analysis. This work was supported by the Max Planck Society for the Advancement of Science and the German Research Foundation (DFG/Gottfried Wilhelm Leibniz Prize). The mass spectrometry proteomics data have been deposited to the ProteomeXchange Consortium via the PRIDE partner repository with the data set PXD003733. References This article references 28 other publications.